The Earth’s Limits and What it Means for the Future of Food
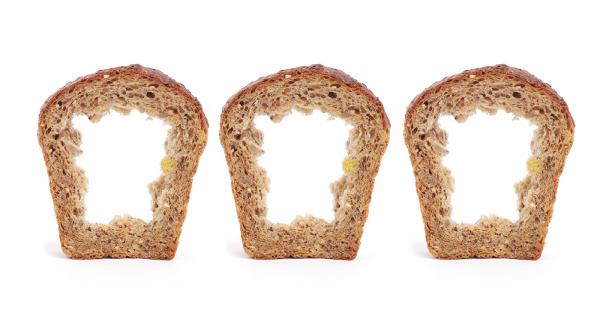
In addition to water, people need food for their very existence. Thus food is also essential to economic growth.
Problems with maintenance of far-flung and intensive food production systems played a role in the collapse of previous civilizations, including the Roman Empire.[1] Mesopotamia, the green and lush center of the Sumerian and Babylonian civilizations, was largely turned to desert as a result of soil erosion. The Mayan civilization likewise succumbed to declining food production, according to recent archaeological research.[2]
Industrial societies have skirted what would otherwise have been limiting factors to food production using irrigation, new crop varieties, fertilizers, herbicides and pesticides, and mechanization — as well as expanded transport networks that allow local abundance to be shared globally. In terms of productivity, twentieth-century agriculture constituted an unprecedented story of success: grain production increased an astounding 500 percent (from under 400 million tons in 1900 to nearly two billion in 2000). This achievement mostly depended on the increasing use of cheap and temporarily abundant fossil fuels.[3]
At the beginning of the twentieth century, most people farmed and agriculture was driven by muscle-power (animal and human). Today in most countries, farmers make up a much smaller proportion of the population than was formerly the case and agriculture is at least partly mechanized. Fuel-fed machines plow, plant, harvest, sort, process, and deliver foods, and industrial farmers typically work larger parcels of land. They also typically sell their harvest to a distributor or processor, who then sells packaged food products to a wholesaler, who in turn sells these products to chains of supermarkets or restaurants. The ultimate consumer of food is thus several steps removed from the producer, and food systems in most nations or regions have become dominated by a few giant multinational seed companies, agricultural chemicals corporations, and farm machinery manufacturers, as well as food wholesalers, distributors, and supermarket and fast-food chains.
Farm inputs have also changed. A century ago, farmers saved seeds from year to year, while soil amendments were likely to come from the farm itself in the form of animal manures. Farmers only bought basic implements, plus some useful materials such as lubricants. Today’s industrial farmer relies on an array of packaged products (seeds, fertilizers, pesticides, herbicides, feed, antibiotics), as well as fuels, powered machines, and spare parts. The annual cash outlays for these can be daunting, requiring farmers to take out substantial loans.
The path to our current food abundance was littered with incidental costs, most borne by the environment. Agriculture has become the single greatest source of human impact upon the planet as a result of soil salinization, deforestation, loss of habitat and biodiversity, fresh water scarcity, and pesticide pollution of water and soil.[4] Fertilizer use worldwide increased 500 percent from 1960 to 2000, and this contributed to an explosion of “dead zones” in seas and oceans, upsetting a process of nutrient cycling that has existed for billions of years.[5]
There have been some environmental improvements to agriculture in recent years: U.S. farming is more energy efficient than it was a couple of decades ago, fertilizer use has declined somewhat, and more effort goes toward soil conservation. But in general, and especially on the global scene, as food production has grown, so have environmental impacts.[6]
Now, further expansion of the food supply appears problematic. World grain production per capita peaked in 1984 at 342 kg annually. For many years production has not met demand, so the gap has been filled by dipping into carryover stocks; currently, less than two months’ supply remains as a buffer.[7]
The challenges to increasing production come from several directions simultaneously: water scarcity (see above), topsoil erosion (we are “mining” topsoil with industrial agriculture at almost four times the rate we are mining coal — over 25 billion tons per year versus 7 billion tons), declining soil fertility, limits to arable land, declining seed diversity, increasing requirements for inputs (pests are developing resistance to common pesticides and herbicides, requiring larger doses), and, not least, increasing costs of fossil fuel inputs.[8]
But as the energy required to run the food system becomes more costly, food is increasingly being used to make energy: Many governments now offer subsidies and other incentives for turning biomass — including food crops — into fuel. This inevitably drives up food prices. Even non-fuel crops such as wheat are affected, as farmers replace wheat fields with more profitable biofuel crops like maize, rapeseed, or soy.
Mineral depletion is also posing a limit to the human food supply. Phosphorus is often a limiting factor in natural ecosystems; that is, the supply of available phosphorus limits the possible size of populations in those environments. That’s because phosphorus is one of the three major nutrients required for plant growth (nitrogen and potassium are the other two). Most agricultural phosphorus is obtained from mining phosphate rock: organic farmers use crude phosphate, while conventional industrial farms use chemically treated forms such as superphosphate, triple superphosphate, or ammonium phosphates. Fortunately, phosphorus can be recycled, as the Chinese did in their traditional food-agriculture systems, where human and animal wastes were returned to the soil. But today vast amounts of what might otherwise be valuable soil nutrients are flushed down waterways, and wind up being deposited at the mouths of rivers.[9]
In 2007, Canadian physicist and agricultural consultant Patrick Déry studied phosphorus production statistics worldwide using Hubbert linearization analysis (a technique used to forecast oil depletion rates) and concluded that the peak of phosphate production has been passed for both the United States (1988) and for the world as a whole (1989). Déry looked at data not only for phosphate that is currently commercially minable, but for reserves of rock phosphate of lower concentrations; he found — no surprise — that these would be more costly to exploit from economic, energetic, and environmental standpoints.[10] Déry’s conclusions are echoed in a recent report by Britain’s Soil Association.[11]
There are three main solutions to the problem of Peak Phosphate: composting of human wastes, including urine diversion; more efficient application of fertilizer; and farming in such a way as to make existing soil phosphorus more accessible to plants.
Food supply challenges extend from farms to the world’s oceans. Fish like cod, sardines, haddock, and flounder have been favorites for decades in Europe and North America, but many of these species are now endangered. Global marine seafood capture peaked in 1994. An international group of ecologists and economists warned in 2006 that the world will run out of wild seafood by 2048 if steep declines in marine species continue at current rates. They noted that as of 2003, 29 percent of all fished species had collapsed, meaning they were at least 90 percent below their historic maximum catch levels. The rate of population collapses continues to accelerate. The lead author of the group’s report, Boris Worm, was quoted as saying, “We really see the end of the line now. It’s within our lifetime. Our children will see a world without seafood if we don’t change things.”[12] According to a more recent study, many types of fish have great difficulty recovering even if over-fishing stops. After 15 years of conservation efforts, many stocks had barely increased in numbers. Cod, for example, failed to recover at all.[13]
Here, then is the overall picture: Demand for food is slowly outstripping supply. Food producers’ ability to meet growing needs is increasingly being strained by rising human populations, falling freshwater supplies, the rise of biofuels industries, expanding markets within industrializing nations for more resource-intensive meat and fish-based diets; dwindling wild fisheries; and climate instability. The result will almost inevitably be a worldwide food crisis sometime in the next two or three decades.[14]
The challenges to increasing global food production or even maintaining current rates are linked not only with the other problems discussed in this chapter (changing climate, energy resource depletion, water scarcity, and mineral depletion) but also with the problems discussed in Chapter 2: Modern agriculture requires a system of credit and debt. Unless farmers can obtain credit, they cannot afford increasingly expensive inputs. Food processors and wholesalers likewise require access to credit. Thus a prolonged credit crisis could devastate the world’s food supply as dramatically as any imaginable weather event.
The solution often proposed to these daunting food system challenges is genetic engineering. If we can splice genes to make more productive crop varieties, more nutritious foods, plants that can grow in saltwater, fish that grow faster, or grains that can fix atmospheric nitrogen the way legumes do, then we could reduce the need for freshwater irrigation, nitrogen fertilizers, and overfishing while growing more food and nourishing people better. It sounds too good to be true — and probably is. In reality, most currently patented plant genes merely confer resistance to insect pests or proprietary herbicides; the promise of more nutrient-rich crops and of nitrogen-fixing grains is still years from realization. Meanwhile, the designer-gene seed industry continues to depend on energy-intensive technologies (such as chemical fertilizers and herbicides), as well as centralized production and distribution systems, along with financial systems based on credit and debt. So far, gene splicing in food plants has succeeded mostly in generating enormous profits for an increasingly centralized corporate seed industry, and more debt for farmers. As for gene-altered fish, ecologists warn that while these are meant to be raised in enclosed areas, if even a few accidentally escaped into the wild they could quickly displace remaining related wild populations and upend fragile and already compromised ecosystems.[15]
It’s worth noting that for the past few decades a vocal minority of farmers, agricultural scientists, and food system theorists including Wendell Berry, Wes Jackson, Vandana Shiva, Robert Rodale, and Michael Pollan, has argued against centralization, industrialization, and globalization of agriculture, and for an ecological agriculture with minimal fossil fuel inputs. Where their ideas have taken root, the adaptation to Peak Oil and the end of growth will be easier. Unfortunately, their recommendations have not become mainstream, because industrialized, globalized agriculture has proved capable of producing larger short-term profits for banks and agribusiness cartels. Even more unfortunately, the available time for a large-scale, proactive food system transition before the impacts of Peak Oil and economic contraction arrive is gone. We’ve run out the clock.
This article is an excerpt from Post Carbon Institute Senior Fellow Richard Heinberg's book The End of Growth, (New Society Publishers, 2011).
Access additional excerpts on the Post Carbon Institute website.
References
1. In his book, Dirt, David Montgomery makes a powerful case that soil erosion was a major cause of the Roman economy’s decline. David Montgomery, Dirt: The Erosion of Civilizations, (Berkeley and Los Angeles: University of California Press, 2007).
2. T. Beach et al., “Impacts of the Ancient Maya on Soils and Soil Erosion in the Central Maya Lowlands,” Catena 65, no.2 (February 28, 2006), 166-178.
3. Richard Heinberg and Michael Blomford, The Food and Farming Transition, Post Carbon Institute, 2009, available online http://www.postcarbon.org/report/41306-the-food-and-farming-transition-toward.
4. Jonathan Foley, “The Other Inconvenient Truth: The Crisis in Global Land Use,” environment 360, posted October 5, 2009.
5. “World Fertilizer Consumption,” spreadsheet for “Food and Agriculture,” Earth Policy Institute Data Center, posted January 12, 2011, http://www.earth-policy.org/data_center/C24; Robert J. Diaz et al., “Spreading Dead Zones and Consequences for Marine Ecosystems,” Science 321, no.926 (2008).
6. David Tilman et al., “Agricultural Sustainability and Intensive Production Practices,” Nature 418 (August 8, 2002), 671-677.
7. Scott Kilman and Liam Pleven, “Harvest Shocker Rattles Wall Street,” The Wall Street Journal, October 9, 2010.
8. Food and Agriculture Organization of the United Nations, “Protect and Produce: Restoring the Land,” in Dimensions of Need–An Atlas of Food and Agriculture (Rome: FAO, 1995); Leo Horrigan, Robert S. Lawrence, and Polly Walker, “How Sustainable Agriculture Can Address the Environmental and Human Health Harms of Industrial Agriculture,” Environmental Health Perspectives 110, no.5 (May, 2002).
9. Patrick Déry and Bart Anderson, “Peak Phosphorus,” The Oil Drum, posted August 17, 2007, www.theoildrum.com/node/2882.
10. Patrick Déry, Pérenniser l’agriculture, Mémoire pour la Commission Sur l’Avenir de l’Agriculture du Québec, GREB, April 2007.
11. A Rock and a Hard Place: Peak Phosphorus and the Threat to our Food Security, (Bristol UK: Soil Association, 2010).
12. Juliet Eilperin, “World’s Fish Supply Running Out, Researchers Warn,” The Washington Post, November 3, 2006.
13. Corinne Podger, “Depleting Fish Stocks,” BBC World Service, posted August 29, 2000.
14. Julian Cribb, The Coming Famine: The Global Food Crisis and What We Can Do to Avoid It, (Berkeley and Los Angeles: University of California Press, 2010).
15. R.D. Howard, J.A. DeWoody, and W.M. Muir, “Transgenic Male Mating Advantage Provides Opportunity for Trojan Gene Effect in Fish,” Proceedings of the National Academy of Science 101, no.9 (February 19, 2004), 2934-2938.